
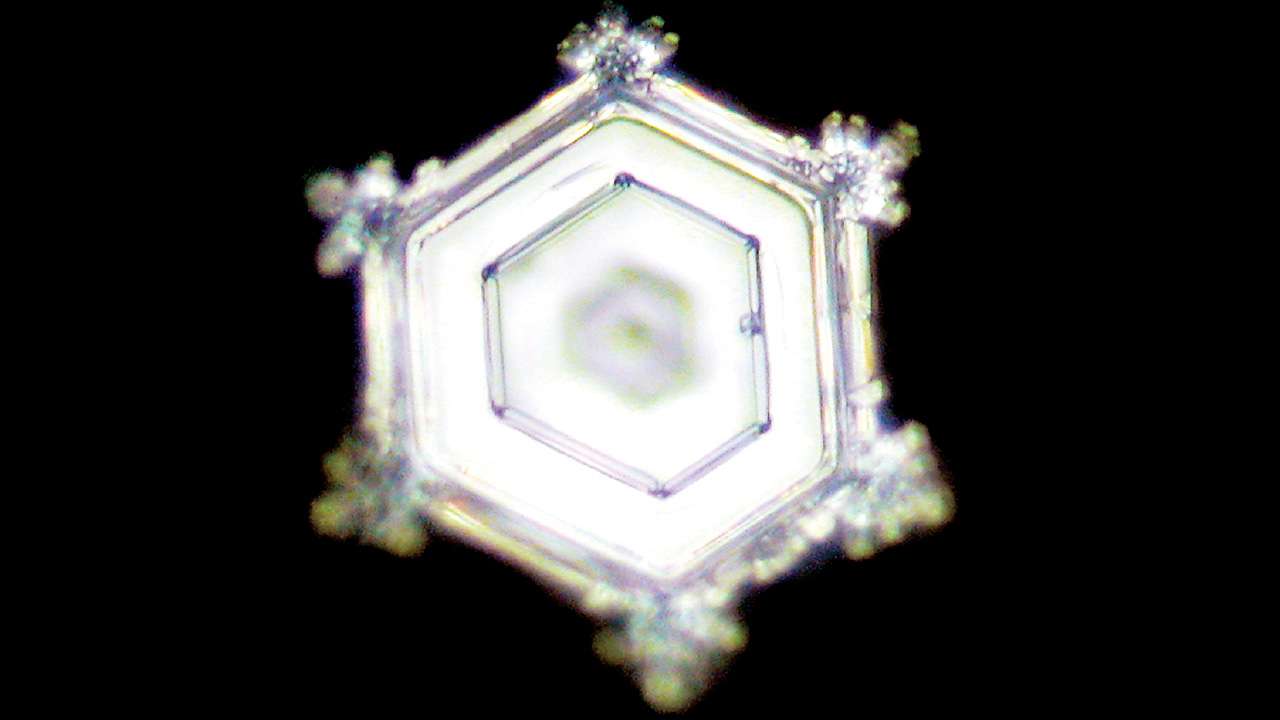
DelloStritto, Maximilian Jaugstetter, Pouya Hosseini, Manuel Corva, Alfred Ludwig, Kristina Tschulik, Michael L. Piontek, Dennis Naujoks, Tadneem Tabassum, Mark J. The Journal of Physical Chemistry B 2023, 127 On the Propensity of Excess Hydroxide Ions at the Alcohol Monolayer–Water Interface.

#Water imoto free
Notwithstanding the interfacial hydrated proton exhibiting bulk-like structures, a substantial interfacial stabilization by −1.3 ± 0.2 kcal/mol is observed experimentally, in good agreement with our free energy calculations. Combined with spectral calculations based on ab initio molecular dynamics simulations, the proton at the water–air interface is shown to be well-hydrated, despite the limited availability of hydration water, with both Eigen and Zundel structures coexisting at the interface. Here, using surface-specific vibrational spectroscopy, we probe the response of interfacial protons at the water–air interface and reveal the interfacial proton continuum. The hydration of the interfacial proton is necessarily different from that in the bulk, given the lower effective density of water at the interface, but has not yet been elucidated. Here, the pressure response is an increase from about 17 to 21 water molecules that solvate the methyl groups despite a sizable radial compression of the hydrophobic solvation shell.Understanding the interfacial molecular structure of acidic aqueous solutions is important in the context of, e.g., atmospheric chemistry, biophysics, and electrochemistry. In the hydrophobic region of TMAO, the solvating water molecules are found to straddle the three methyl groups at ambient pressure, which remains virtually unchanged upon compressing the solution to 10 kbar. Moreover, only a negligible contribution of non-H-bonded water molecules is found in the first-shell region of TMAO even at 10 kbar, in contrast to the pressure response of water itself. Under ambient conditions, the negatively charged oxygen site of the solute, which is strongly hydrophilic, predominantly accepts three H-bonds, whereas a roughly equal population of threefold and square-planar fourfold H-bonding is observed at 10 kbar. The pressure response of TMAO(aq) is distinctly different, although its radial distribution functions do not change much.
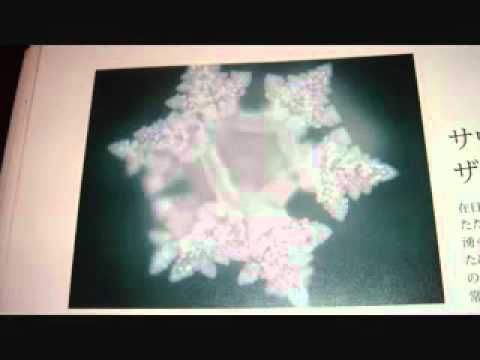
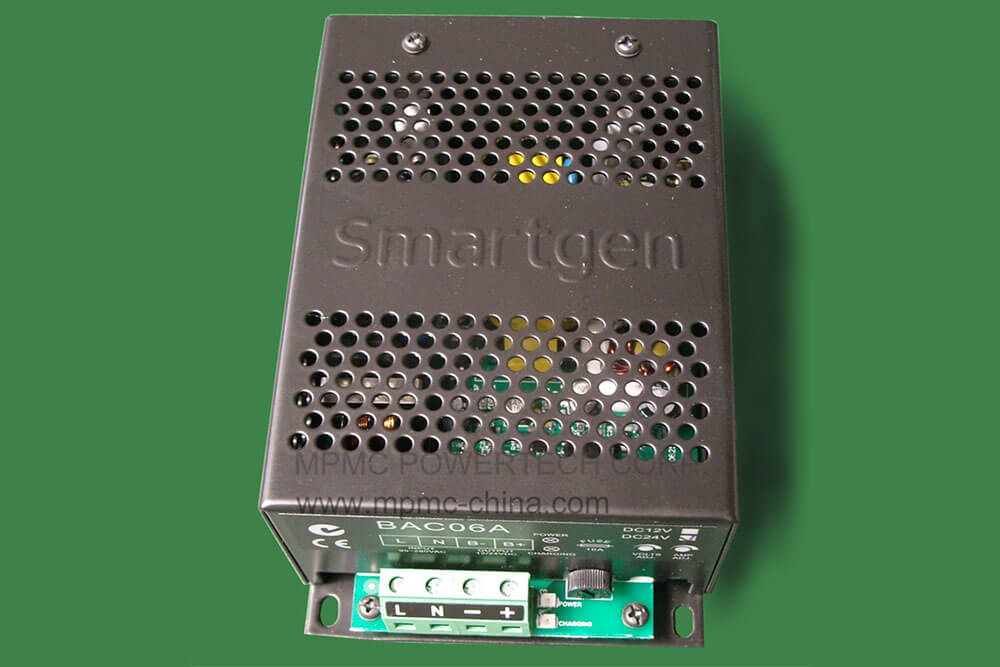
These additional molecules increase the coordination number of pure water at 10 kbar significantly, but they are definitely not H-bonded to the central water molecule rather they are its topological second to fourth H-bonded neighbors. The eye-catching shape changes of its oxygen–oxygen radial distribution function, where apparently the entire second peak is shifted into the first one, are traced back to about two more water molecules which are squeezed into the tetrahedral voids that are formed in the first shell by the H-bonded water molecules. Pure water is shown to prefer to keep four H-bonded water molecules in a locally tetrahedral arrangement up to 10 kbar. The neat solvent, liquid water compressed to 10 kbar, is analyzed in detail to provide a meaningful gauge for the pressure-induced solvation changes of the solute. Here, using ab initio molecular dynamics, we analyze how its solvation structure changes upon compressing its ≈0.5 M aqueous solution from 1 bar to 10 kbar. Yet, even the solvation of TMAO itself is not well understood beyond ambient conditions. Trimethylamine N-oxide (TMAO) is a protecting osmolyte that stabilizes proteins against both temperature and pressure denaturation.
